San Diego, California, USA
March 20, 2015
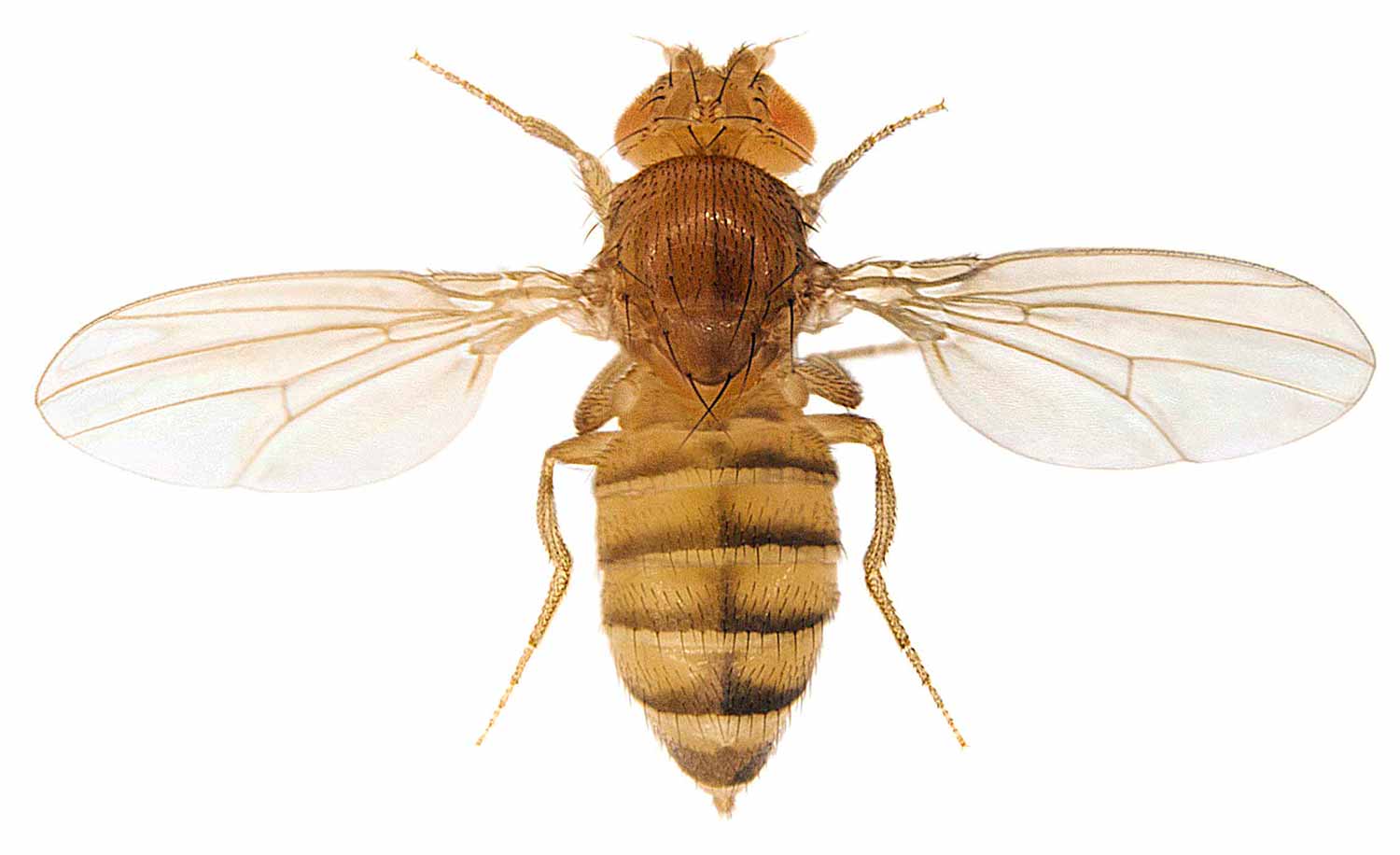
A rare fruit fly in which the left half has been mutated by MCR (hence the pale color) while the right half remains normal. Credit: Valentino Gantz, UC San Diego
Biologists at the University of California, San Diego have developed a new method for generating mutations in both copies of a gene in a single generation that could rapidly accelerate genetic research on diverse species and provide scientists with a powerful new tool to control insect borne diseases such as malaria as well as animal and plant pests.
Their achievement was published today in an advance online paper in the journal Science. It was accomplished by two biologists at UC San Diego working on the fruit fly Drosophila melanogaster who employed a new genomic technology to change how mutations could spread through a population—a concept long established in plants by the father of modern genetics, Gregor Mendel.
“Mendel conducted classic genetic experiments with peas that revealed the fundamental of inheritance in many organisms including humans,” explains Ethan Bier, a professor of biology at UC San Diego whose graduate student, Valentino Gantz, developed the method. “According to these simple rules of inheritance, the fertilized egg receives one copy of most genes from our mothers and one from our fathers so that the resulting individual has two copies of each gene.”
One advantage of having two copies of a gene is that if one copy carries a non-functional mutation, then the other “good” copy typically can provide sufficient activity to sustain normal function. Thus, most mutations resulting in loss of gene function are known as recessive, meaning that an organism must inherit two mutant copies of the gene from its parents before an overt defect is observed, as is the case in humans with muscular dystrophy, cystic fibrosis or Tay Sachs disease.
“Because individuals carrying a single mutant copy of a gene often mate with an individual with two normal copies of gene, defects can be hidden for a generation and then show up in the grandchildren,” Bier adds. “This is how genetics has been understood for over a century in diverse organisms including humans, most animals we are familiar with, and many plants.”
But in the past two years, Bier and other molecular biologists have witnessed a veritable revolution in genome manipulation. “It is now routine to generate virtually any change in the genome of an organism of choice at will,” he notes. “The technology is based on a bacterial anti-viral defense mechanism known as the Cas9/CRISPR system.”
By employing this development in their experiments with laboratory fruit flies, Gantz and Bier demonstrated that by arranging the standard components of this anti-viral defense system in a novel configuration, a mutation generated on one copy of a chromosome in fruit flies spreads automatically to the other chromosome. The end result, Bier says, is that both copies of a gene could be inactivated “in a single shot.”
The two biologists call their new genetic method the “mutagenic chain reaction,” or MCR.
“MCR is remarkably active in all cells of the body with one result being that such mutations are transmitted to offspring via the germline with 95 percent efficiency,” says Gantz, the first author of the paper. “Thus, nearly all gametes of an MCR individual carry the mutation in contrast to a typical mutant carrier in which only half of the reproductive cells are mutant.”
Bier says “there are several profound consequences of MCR. First, the ability to mutate both copies of a gene in a single generation should greatly accelerate genetic research in diverse species. For example, to generate mutations in two genes at once in an organism is typically time consuming, because it requires two generations, and involved, because it requires genetic testing to identify rare progeny carrying both mutations. Now, one should simply be able to cross individuals harboring two different MCR mutants to each other and all their direct progeny should be mutant for both genes.”
“MCR should also be highly effective for dispersing genetic elements in populations to control insect borne diseases such as malaria, dengue and chikungunya as well as animal and plant pests,” he adds. “For example, in the case of malaria, several groups have created genetic cassettes that when introduced into mosquitoes prevent the malarial parasite from propagating thereby blocking infection. A major challenge in the field, however, has been devising a way to disseminate these gene cassettes throughout mosquito populations. MCR offers an obvious solution to this problem since the incorporation of an anti-malarial gene cassette into an MCR element should result in the rapid spread of the gene cassette through the target population. For example, if one in 100 individuals initially carried the cassette, the cassette should spread to virtually all individuals in as few as 10 generations, which is less than one season for mosquitoes.”
It may also be possible to use MCR to spread genes among cells within an individual using modified viruses to carry the genetic elements, Gantz points out. “Since MCR works by targeting specific DNA sequences, in cases where diseased cells have altered DNA as in HIV-infected individuals or some types of cancer, MCR-based methods should be able to distinguish diseased from healthy cells and then be used to selectively either destroy or modify the diseased cells.”
The two biologists note in their paper that while applications of MCR offer potential solutions to important problems in health and human welfare, it could also pose serious potential risks in the wrong hands.
“Could an accidental release of MCR-bearing organisms into the environment result in their spreading potentially deleterious mutation to the vast majority of individuals in a wild population?” says Bier. “We don’t know and would advocate that scientists examine this possibility before permitting experiments using the new method to be used in open laboratories. It is also possible that MCR technology could be intentionally misused, which should be considered as a risk on par with that associated with nefarious uses of select agents.”
Gantz and Bier showed in their experiments, which were conducted in a biosafety laboratory with a high-level of containment to prevent the accidental release of any deleterious mutant genotypes, that propagation of an MCR mutation in flies is remarkably efficient, with a gene conversion rate of about 95 percent.
As a consequence, the two researchers say stringent safety protocols for handling MCR organisms and the prompt establishment of regulatory guidelines for performing experiments with such organisms are imperative. “Development of methods for reversing MCR spread would also provide a means for mitigating risk,” says Gantz.
Bier concurs with others who have raised serious concerns about strategies to disperse genetic elements in pest populations that one other step needs to be taken by scientists. “In an analogy to the famous Asilomar conference concerns held to address concerns raised at the dawn of recombinant DNA technology in the 1970s,” he says, “perhaps a similar meeting should be convened to discuss how MCR technology should be regulated at both federal and institutional levels to assure that it is employed safely to achieve its full potential to ameliorate the human condition.”
The study was funded by two grants (R01 GM067247 and R56 NS029870) from the National Institutes of Health and a generous gift from Sarah Sandell and Michael Marshall. The UC San Diego Technology Transfer Office has applied for a patent to license the new technology.